LAB REPORT
Science and Technology Making Headlines
July 17, 2020
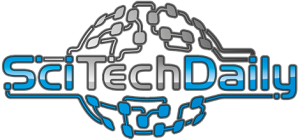
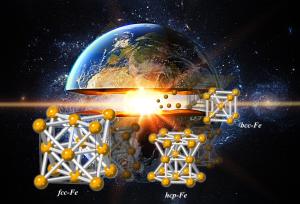
Usually, iron atoms are arranged with one atom at the center of a cube and at each vertex (right). At high enough temperatures, the iron maintains this cubic arrangement with iron atoms at each vertex, but rather than one iron atom in the center there is an iron atom at each of the six cube faces (left). At high enough pressures, the arrangement of iron atoms transforms from a cube into a hexagonal pattern (center). Credit: Yonsei University
Heavy metal to the core
New research by Lawrence Livermore researchers and collaborators could offer insights into the formation of planets like Earth and inform the design of more resilient materials.
Iron is the most abundant metal in the universe. It forms the complex structure at Earth’s core, producing a planetary magnetic field that protects life on its surface from space radiation.
To understand how iron and other materials act in the extreme conditions of planetary cores, scientists use powerful laser beam pulses to launch a shockwave into a material and increase its pressure and temperature.
Now, an international team of scientists has observed how the structure of iron changes during a rapid impact produced by a laser pulse. The way iron atoms rearrange in response to this extreme shock offers new insights into the formation of planets like Earth.

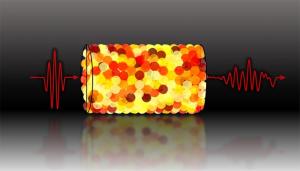
The image is a combination of two sets of data from X-ray scans of single crystal sapphire spheres. The combination and colorization of this data shows the distribution of stresses for each grain under load. This information was used as initial conditions for ultrasonic transmission measurements.
All stressed out
Better understanding of stress wave propagation through grainy materials is important for detecting the magnitude of earthquakes, locating oil and gas reservoirs and designing acoustic insulation and materials for compacting powders.
A team of researchers including Lawrence Livermore physicist Eric Herbold used X-ray measurements and analyses to show that velocity scaling and dispersion in wave transmission is based on grainy particle arrangements and chains of force between them, while reduction of wave intensity is caused mainly from grainy particle arrangements alone.
The structure-property relations of granular materials are governed by the arrangement of particles and the chains of forces between them. These relations allow the design of wave-damping materials and non-destructive testing technologies. Wave transmission in granular materials has been extensively studied and demonstrates unique features, including power-law velocity scaling, dispersion and attenuation (the reduction of the amplitude of a signal, electric current or other oscillation).
Earlier research, dating back to the late 1950s, described "what" may be happening to the material underlying wave propagation, but the new research provides evidence for "why."
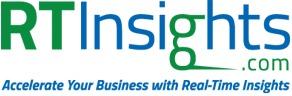
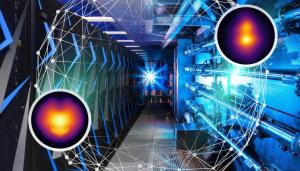
Researchers are integrating technologies such as the Sierra supercomputer (left) and the National Ignition Facility (right) to understand complex problems like fusion in energy and the aging effects in nuclear weapons. Data from NIF experiments (inset, right) and simulations (inset, left) are being combined with deep learning methods to improve areas important to national security and our future energy sector. Illustration by Tanya Quijalvo/LLNL.
Surrogates are better than simulators
Besides time and cost savings, surrogate models could lead to new scientific discoveries because of their ability to handle large volumes of high-dimensional data.
New research from Lawrence Livermore demonstrated deep learning surrogate models performing as well, and sometimes better, than more expensive simulators.
The researchers tested the surrogate model on complicated inertial confinement fusion problems and found it could accurately emulate scientific processes, while also reducing compute time from half an hour to a few seconds.
The new deep learning-driven model significantly outperformed current surrogate models, while adequately replicating the simulator results.
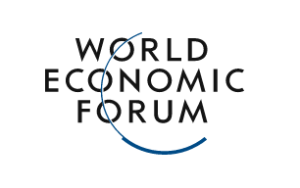
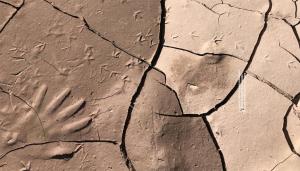
LLNL scientists used fingerprinting — the process of separating the relative roles of natural and human influences on global climate — to identify how greenhouse gas and particulate pollution emissions drive regional drying around the globe.
Fingerprints point to globe running dry
Human-caused climate change has intensified patterns of extreme rainfall and drought across the globe, according to a new study by Lawrence Livermore scientists.
Increasing greenhouse emissions and aerosols have been major contributing factors to drought patterns through the industrial era. To understand the true extent of the problem, scientists have used a new technique called fingerprinting — the process of separating the relative roles of natural and human influences on global climate
The study finds there is a detectable “human fingerprint” on decreasing rainfall over the U.S, Central Asia and Southern Africa, according to the results It is also detectable on increasing rainfall in the Sahel region of Africa, India and the Caribbean.
In addition to increasing greenhouse gas emissions, aerosols released by human pollution and large volcanic eruptions also have been “major contributing agents” to global drought patterns through the industrial era, the research says.
The findings “tackle the problem of identifying the human influence of drought patterns across the world,” said LLNL climate scientist Celine Bonfils.
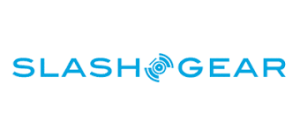
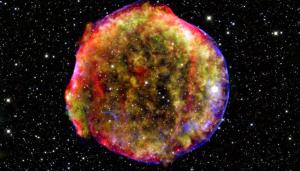
This image of the Tycho supernova remnant, captured by NASA's Spitzer and Chandra space observatories and the Calar Alto observatory in Spain, combines infrared and X-ray observations. Image courtesy of MPIA/NASA/Calar Alto Observatory.
Miming the cataclysmic death of a star
When stars explode into supernovae, they produce shock waves in the plasma surrounding them. Scientists say that the shock waves are so powerful they can act as particle accelerators that blast streams of particles, called cosmic rays, into the universe at nearly the speed of light. One of the mysteries of science is how exactly the supernovae do that.
Lawrence Livermore scientists and collaborators have devised a new way to study the workings of this sort of astrophysical shock wave by creating a scaled-down version of the shock in the lab.
The scientists found that the astrophysical shocks develop turbulence at small scales that can’t be seen by observations. That turbulence helps kick electrons toward the shock wave before they are boosted to their final and extremely high speed. Researchers say that while the mechanics at play are fascinating, they’re so far away that it’s hard to study them.
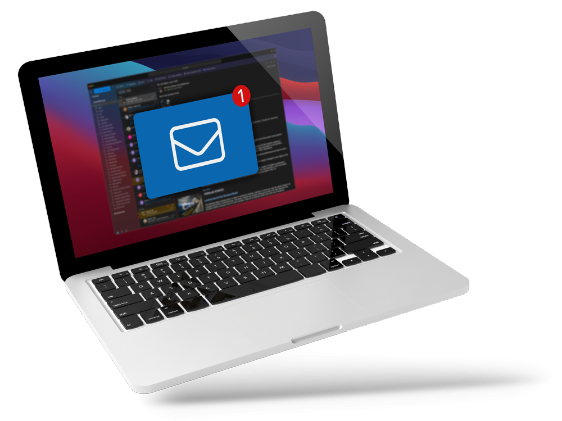