LAB REPORT
Science and Technology Making Headlines
March 5, 2021

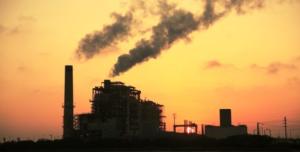
LLNL scientists have offered a suite of suggestions to get California and ultimately the nation carbon neutral by 2045.
The green light on carbon neutrality
California is well positioned to help the United States meet President Biden’s nationwide carbon neutrality goal.
And it comes down to engineered solutions. While most of the required greenhouse gas reductions will come from clean technology and traditional emission reduction programs, the United Nations Intergovernmental Panel on Climate Change has made it clear that meeting these targets also will necessitate actively removing substantial amounts of carbon already in the atmosphere, as well as preventing new carbon emissions from reaching the atmosphere.
A 2020 report by Lawrence Livermore National Laboratory concluded that California’s carbon neutrality goals will require the state to reach negative emissions through a combination of engineered technologies and nature-based solutions like sequestration in soils and plants.
First, the state has a long history of setting — and achieving — ambitious climate goals, including emission reduction and renewable energy targets met in 2020, and more recently a goal of achieving statewide carbon neutrality by 2045. Second, California has led the deployment of similar emission reduction technology to great success.
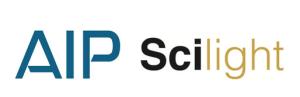
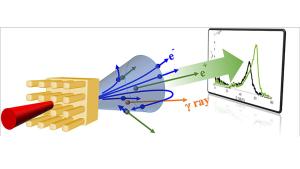
Creating antimatter in the laboratory can help researchers gain insight into gamma ray bursts. Image courtesy of NASA.
Billions and billions of antimatter particles
Creating antimatter (also known as positrons) in a small laboratory setting has attracted great interest from researchers. It helps them understand the physics underlying various astrophysical phenomena, such as black holes and gamma ray bursts, and paves the way for creating a dense electron-positron plasma in the laboratory. Making enough electron-positron antimatter for laboratory astrophysics applications, however, is usually considered beyond the capability of state-of-the-art lasers.
Lawrence Livermore scientists provide clear experimental and simulation results demonstrating a substantial increase in the yield and energy of positrons, produced by high-intensity lasers, by using large-scale, front-surface target structures.
By simply adding microstructures to the target and without having to upgrade the laser, the researchers’ microwire array target yielded a near 100 percent increase in the laser-to-positron conversion efficiency and a 10 megaelectronvolt increase in positron energy. Earlier results using a simpler target created 1 billion particles of antimatter.
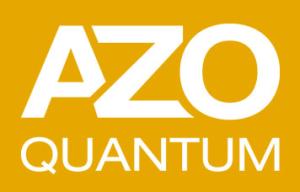
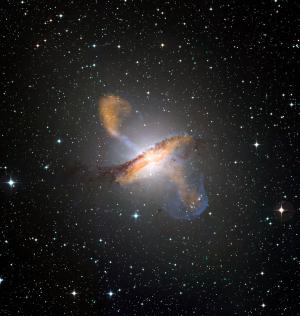
Researchers have determined that magnetic fields have a dramatic effect on the shape of astrophysical jets like these powered by a black hole. Image courtesy of NASA's Goddard Space Flight Center.
Jetting off in different directions
Matter outflows are common attributes arising from systems driven by compact objects like active galactic nuclei, black holes, accreting objects like Young Stellar Objects (YSO), pulsar wind nebulae and mature stars like the sun.
However, the shape of such outflows, also called astrophysical jets, differ based on the magnetic field surrounding them.
New experiments by a scientist from Lawrence Livermore and international collaborators reveal that outflow/magnetic field misalignment is a viable key process that regulates jet formation.
The researchers used a high powered laser at the École Polytechnique to generate fast material outflows in a powerful applied magnetic field as a surrogate for prospective astrophysics conditions. They particularly analyzed how the jet formation is influenced by a misalignment between where the jet initially forms and then the magnetic field.
In the case of minor misalignments, a magnetic nozzle is formed, which redirects the outflow in a parallel jet. In the case of major misalignments, this nozzle turns more and more asymmetric, thus interrupting jet formation.
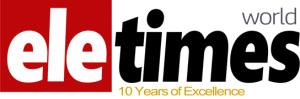
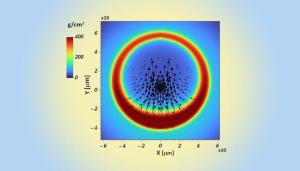
This is a HYDRA simulation of an ICF implosion with an imposed HDC shell thickness asymmetry. The result plotted here is a significantly distorted shell at peak compression (background color scale) along with the induced hotspot flow field. Image by Chris Schroeder and Jose Milovich/LLNL.
The tiniest thing can have big consequences
Inertial Confinement Fusion (ICF) implosions require very high levels of symmetry to reach the high densities and temperatures required for fusion-induced self-heating. Even percent-level deviations from perfect spherical symmetry can lead to significant distortions of the implosion and ultimately degrade fusion performance.
To that end, researchers from Lawrence Livermore National Laboratory conducted work to gain a better understanding of why this happens.
Daniel Casey, Laboratory physicist and lead author of the paper, said the work summarizes observations of areal-density asymmetries seeded by high-density carbon-capsule thickness asymmetries, helping to illuminate one of the principal causes of a significant degradation in ICF implosions at the National Ignition Facility, the world’s most energetic laser.
“These asymmetries can decrease the energy available to heat the hotspot and reduce the confinement of that energy,” Casey said. “It is like squeezing a balloon a little harder on one side than the other -- at some point the balloon will attempt to vent out the weak spots.”
The research reveals that tiny imperfections in the capsule can grow into huge distortions of the implosion at peak compression.

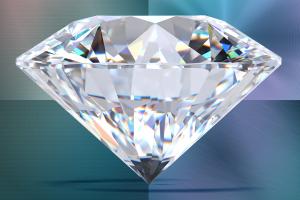
Diamond remains stable under extreme pressure.
Rock in a hard place
Diamond is surprisingly good under pressure. Its crystal structure holds up even when compressed to more than five times the pressure in Earth’s core.
Lawrence Livermore scientists found that diamond isn’t always carbon’s most stable structure unless it's under pressure. Pure carbon can take on many forms. Diamond is one. Others include graphite (found in pencil lead) and tiny, cylinder shapes called carbon nanotubes. Carbon’s atoms are arranged in different ways for each form. Those patterns can be more or less stable under different conditions. Usually, carbon atoms take on the most stable state possible.
At normal pressures on Earth’s surface, carbon’s most stable state is graphite. But given a forceful squeeze, diamond wins out. That’s why diamonds form after carbon takes a plunge inside Earth.
But at even higher pressures, scientists had predicted that new crystal structures would be more stable than diamond. LLNL physicist Amy Lazicki and colleagues pummeled diamond with powerful lasers. Then they used X-rays to measure the material’s structure. The predicted new crystals never showed up. Diamond persisted even after this laser beating.
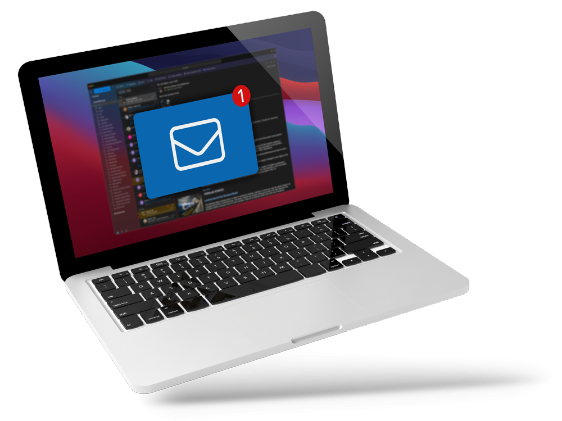