LAB REPORT
Science and Technology Making Headlines
June 18, 2021

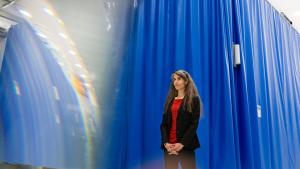
LLNL’s Federica Coppari stands inside the National Ignition Facility’s laser bay.
What’s going on up there
Out in the vast universe, unknown billions of strange worlds drift around other stars. Many of them are quite unlike anything in our solar system. While astronomers hope to use immense upcoming observatories to get a better look at their outsides, Lawrence Livermore’s Federica Coppari has been using the world’s largest laser to investigate their insides.
Coppari compresses familiar substances, including rocks and water, into new forms. Her work has yielded insights into the inner workings of frozen giants such as Uranus and Neptune, as well as the potential habitability of super-Earths — rocky planets that dwarf our own.
Two years ago, she and her colleagues confirmed the existence of “superionic ice,” a hot, black form of the normally chilly substance. This long-sought phase may be among the most common forms of ice in the universe, found inside ice giants both in our own solar system and elsewhere. The work has helped solve puzzles about such worlds’ magnetic fields.
It’s here, where the terrestrial and the celestial meet, that Coppari thrives. “How our experiments in the laboratory can say something about what’s going on up there — that’s fascinating,” she said.
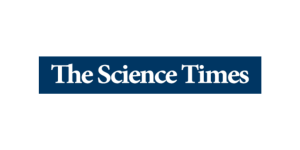
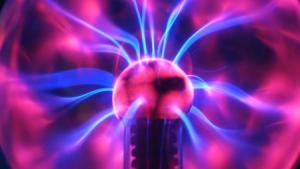
Scientists have created plasma, also known as a fourth state of matter, in the laboratory.
Probing a fourth state of matter
Possibly the most extreme and rare states of matter on Earth are laser-produced high-density energy plasmas. These forms of matter are equal to those found in nuclear explosions, giant planet cores and stars.
After nearly a decade of collaboration between the Department of Energy (DOE) and Lawrence Livermore's National Ignition Facility (NIF), scientists from DOE's Princeton Plasma Physics Laboratory have built a novel X-ray crystal spectrometer that would be able to provide clear, high-resolution measurements of HED plasmas produced by NIF.
NIF is home to the largest and most powerful lasers in the globe. The decade-long collaboration is a significant expansion on Princeton Plasma Physics Laboratory's X-ray crystal spectrometer designs that are used in fusion laboratories worldwide.
NIF laser-produced high-density plasmas are minuscule, point-like substances that require high-definition spectrometers designed to enable high-resolution studies.
The novel designs differ from the current magnetically confined experiments being conducted in the Princeton Plasma Physics Laboratory. One key difference is the tiny size of laser-produced High Energy Density (HED) plasmas that typically have a volume of one cubic millimeter, which can be considered the point-like X-ray source.

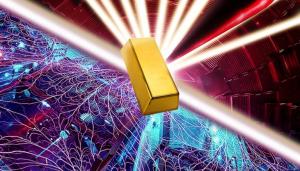
Using the world’s most energetic laser and the world’s most powerful pulsed-power facility, an international research team has derived new pressure scales for gold and platinum at 1 terapascal.
Gold crush
Robust new calibration standards for studying materials at extreme pressures have been developed by researchers in the United States. Dayne Fratanduono of Lawrence Livermore and colleagues used dynamic compression experiments to establish clear relationships between the pressures and volumes of both gold and platinum at terapascal (TPa) pressures. These standards could better coordinate the findings of independent high-pressure experiments and theoretical predictions.
Terapascal pressures are tens of million times greater than atmospheric pressure and have profound effects on structures of atoms and the intermolecular forces that hold materials together. Indeed, the quantum mechanical pressure that prevents an electron from being sucked into an atomic nucleus is in the terapascal range.
Such pressures can transform gases such as hydrogen, oxygen and xenon into solid metals and even make some materials room-temperature superconductors. Terapascal pressures can be found at the centers of giant planets – with Jupiter having core pressures as high as 7 TPa. As a result, the ability to reliably study materials at terapascal pressures in the lab benefits astronomers and materials scientists alike.
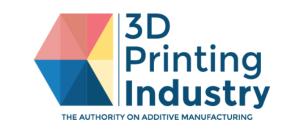
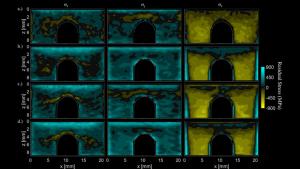
A research team has found that island scanning, a printing scheme commonly used to prevent stresses from degrading or destroying 3D-printed metal parts, may not be a cure after all.
Stressed out on an island
New research from Lawrence Livermore National Laboratory (LLNL) suggests that a well-established method of reducing residual stresses in metal 3D-printed parts may not be as effective as the additive manufacturing sector thinks it is.
Island scanning — a common laser scan strategy — is often used by manufacturers to de-stress metal parts 3D printed via laser powder bed fusion. The approach involves partitioning the layers of a build into smaller sub-sections, usually in the shape of squares, to reduce contractions in the part as it is 3D printed.
The team, comprising scientists from LLNL and the National Institute of Standards and Technology (NIST and other institutions, found that the island scanning method actually increased residual stresses in certain bridge-like geometries.
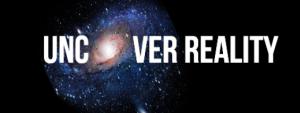
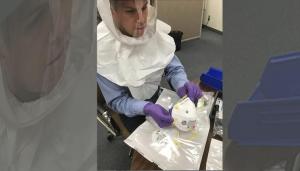
LLNL scientist Kyle Fuhrer prepares an N95 mask for a fit test. Photo by Sam Paik/LLNL.
A snug fit
Scientists at Lawrence Livermore National Laboratory (LLNL) have determined that heating N95 respirators up to 75 degrees Celsius for 30 minutes deactivates a surrogate coronavirus without compromising the device’s fit and its ability to filter airborne particles.
This temperature (equivalent to 167 degrees Fahrenheit) is easily achieved in hospitals and field settings allowing for the N95s to be reused once decontaminated. This heat treatment can be applied at least 10 times on an N95 respirator without degrading its fit. Previous studies have reported that the filtration efficiency of N95s is not negatively impacted by these heating conditions.
“These results suggest that thermal inactivation of coronaviruses is a potentially rapid and widely deployable method to reuse N95 respirators in emergency situations where reusing the respirators is necessary and sterilization is unavailable,” said LLNL electrical engineer Travis Massey, lead author of the paper.
N95 respirators are protective devices that filter airborne particles. The N95 designation means that the respirator blocks at least 95 percent of very small (0.3 micrometers or larger) test particles. They are typically used a single time in health care settings because the respirators can be contaminated when treating infected patients, thereby posing a risk to caregivers who continue wearing a contaminated device, as well as other patients treated by the provider. Limited supply and crisis conservation strategies made reuse a common practice.
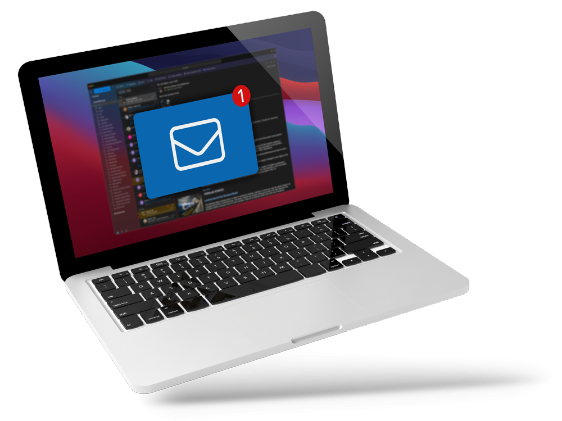