First experimental evidence for superionic ice
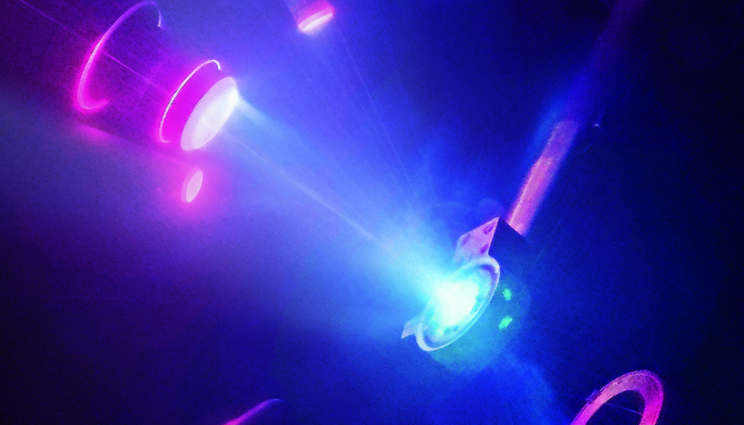
Among the many discoveries on matter at high pressure that garnered him the Nobel Prize in 1946, scientist Percy Bridgman discovered five different crystalline forms of water ice, ushering in more than 100 years of research into how ice behaves under extreme conditions.
One of the most intriguing properties of water is that it may become superionic when heated to several thousand degrees at high pressure, similar to the conditions inside giant planets like Uranus and Neptune. This exotic state of water is characterized by liquid-like hydrogen ions moving within a solid lattice of oxygen.
Since this was first predicted in 1988, many research groups in the field have confirmed and refined numerical simulations, while others used static compression techniques to explore the phase diagram of water at high pressure. While indirect signatures were observed, no research group has been able to identify experimental evidence for superionic water ice -- until now.
In a paper published today by Nature Physics, a research team from Lawrence Livermore National Laboratory (LLNL), the University of California, Berkeley and the University of Rochester provides experimental evidence for superionic conduction in water ice at planetary interior conditions, verifying the 30-year-old prediction.
Using shock compression, the team identified thermodynamic signatures showing that ice melts near 5000 Kelvin (K) at 200 gigapascals (GPa -- 2 million times Earth’s atmosphere) -- 4000 K higher than the melting point at 0.5 megabar (Mbar) and almost the surface temperature of the sun.
"Our experiments have verified the two main predictions for superionic ice: very high protonic/ionic conductivity within the solid and high melting point," said lead author Marius Millot, a physicist at LLNL. "Our work provides experimental evidence for superionic ice and shows that these predictions were not due to artifacts in the simulations, but actually captured the extraordinary behavior of water at those conditions. This provides an important validation of state-of-the-art quantum simulations using density-functional-theory-based molecular dynamics (DFT-MD)."
"Driven by the increase in computing resources available, I feel we have reached a turning point," added Sebastien Hamel, LLNL physicist and co-author of the paper. "We are now at a stage where a large enough number of these simulations can be run to map out large parts of the phase diagram of materials under extreme conditions in sufficient detail to effectively support experimental efforts."
Using diamond anvil cells (DAC), the team applied 2.5 GPa of pressure (25 thousand atmospheres) to pre-compress water into the room-temperature ice VII, a cubic crystalline form that is different from "ice-cube" hexagonal ice, in addition to being 60 percent denser than water at ambient pressure and temperature. They then shifted to the University of Rochester’s Laboratory for Laser Energetics (LLE) to perform laser-driven shock compression of the pre-compressed cells. They focused up to six intense beams of LLE’s Omega-60 laser, delivering a 1 nanosecond pulse of UV light onto one of the diamonds. This launched strong shock waves of several hundred GPa into the sample, to compress and heat the water ice at the same time.
"Because we pre-compressed the water, there is less shock-heating than if we shock-compressed ambient liquid water, allowing us to access much colder states at high pressure than in previous shock compression studies, so that we could reach the predicted stability domain of superionic ice," Millot said.
The team used interferometric ultrafast velocimetry and pyrometry to characterize the optical properties of the shocked compressed water and determine its thermodynamic properties during the brief 10-20 nanosecond duration of the experiment, before pressure release waves decompressed the sample and vaporized the diamonds and the water.
"These are very challenging experiments, so it was really exciting to see that we could learn so much from the data -- especially since we spent about two years making the measurements and two more years developing the methods to analyze the data," Millot said.
This work also has important implications for planetary science because Uranus and Neptune might contain vast amount of superionic water ice. Planetary scientists believe these giant planets are made primarily of a carbon, hydrogen, oxygen and nitrogen (C-H-O-N) mixture that corresponds to 65 percent water by mass, mixed with ammonia and methane.
Many scientists envision these planets with fully fluid convecting interiors. Now, the experimental discovery of superionic ice should give more strength to a new picture for these objects with a relatively thin layer of fluid and a large "mantle" of superionic ice. In fact, such a structure was proposed a decade ago -- based on dynamo simulation -- to explain the unusual magnetic fields of these planets. This is particularly relevant as NASA is considering launching a probe to Uranus and/or Neptune, in the footsteps of the successful Cassini and Juno missions to Saturn and Jupiter.
"Magnetic fields provide crucial information about the interiors and evolution of planets, so it is gratifying that our experiments can test -- and in fact, support -- the thin-dynamo idea that had been proposed for explaining the truly strange magnetic fields of Uranus and Neptune," said Raymond Jeanloz, co-author on the paper and professor in Earth & Planetary Physics and Astronomy at the University of California, Berkeley. It’s also mind-boggling that frozen water ice is present at thousands of degrees inside these planets, but that’s what the experiments show."
"The next step will be to determine the structure of the oxygen lattice," said Federica Coppari, LLNL physicist and co-author of the paper. "X-ray diffraction is now routinely performed in laser-shock experiments at Omega and it will allow to determine experimentally the crystalline structure of superionic water. This would be very exciting because theoretical simulations struggle to predict the actual structure of superionic water ice."
Looking ahead, the team plans to push to higher pre-compression and extend the technique to other materials, such as helium, that would be more representative of planets like Saturn and Jupiter.
Co-authors include Hamel, Peter Celliers, Coppari, Dayne Fratanduono, Damian Swift and Jon Eggert from LLNL; Jeanloz from UC Berkeley; and Ryan Rygg and Gilbert Collins, previously at LLNL and now at the University of Rochester. The experiments also were supported by target fabrication efforts by LLNL’s Stephanie Uhlich, Antonio Correa Barrios, Carol Davis, Jim Emig, Eric Folsom, Renee Posadas Soriano, Walter Unites and Timothy Uphaus.
Contact
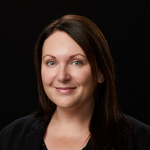
[email protected]
(925) 423-9802
Related Links
Nature PhysicsTags
Lasers and Optical S&TLasers
National Ignition Facility and Photon Science
Physical and Life Sciences
Featured Articles

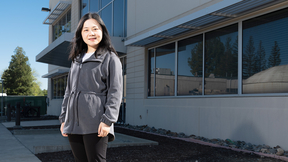
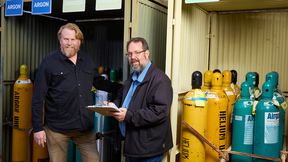