LAB REPORT
Science and Technology Making Headlines
April 8, 2022


A gold cylinder known as a hohlraum holds a fuel capsule at its center for fusion experiments at the National Ignition Facility. Target-handling systems precisely position the capsule and cool it to cryogenic temperatures.
Burning for you
Inertial fusion requires a thousand-fold compression of matter to ultrahigh densities and temperatures. The sun and other stars use gravity to do the job and fuse hydrogen into helium. To mimic the effect on Earth, scientists at Lawrence Livermore National Laboratory’s National Ignition Facility (NIF) use the world’s most powerful bank of lasers to squeeze isotopes of hydrogen — deuterium and tritium — in a 2-mm-wide capsule.
The facility trains 192 laser beams into a 1-cm-tall, hollow, gold-lined cylinder known as a hohlraum that suspends the capsule inside it. After absorbing UV-laser light, the hohlraum’s interior wall reradiates a flux of soft X-rays. Within 8 nanoseconds, those X-rays accelerate and compress the hydrogen isotopes into a hot spot half the width of a human hair at a temperature of 60 million kelvin and a pressure of 350 GPa (one GPa is equivalent to 9,869 atmospheres of pressure on Earth).
Under the capsule’s surface the hydrogen fuel resides as a thin shell, cooled to 18 K prior to compression. The colder the fuel is initially, the more compressible it is — and hence the hotter and denser it becomes. The fuel’s own inertia provides enough delay between the implosion and its sudden deceleration for the strong nuclear force to convert a small fraction of isotope pairs into neutrons and helium nuclei, or alpha particles.


The multiscale model incorporates both microstructural (left) and atomistic (right) simulations to understand barriers to ion transport in solid-state battery materials. Image by Brandon Wood, Tae Wook Heo and Sabrina Wan/LLNL.
Breaking down barriers
Solid electrolytes may overcome key technological hurdles associated with the narrow electrochemical and thermal stability of conventional lithium (Li)-ion and sodium (Na)-ion batteries.
However, many solid electrolytes — ceramics in particular — also suffer from poor cycling issues and limitations in their ability to efficiently transport ions. These limitations often stem from interfaces and other features that make up the microstructure of the material, which in turn depends on how it is processed.
Lawrence Livermore National Laboratory scientists in collaboration with San Francisco State University and Penn State have developed a broad suite of multiscale simulation capabilities to help identify, assess and overcome microstructural impacts on ion transport in solid electrolytes


A cryogenic target used for experiments producing burning-plasma conditions. Photo by Jason Laurea/LLNL.
The search for the holy grail
If you’ve ever been outside during the day, you’ve seen a fusion reactor — though you’re not supposed to stare directly at it. The sun is our fusion reactor in the sky. It’s constantly fusing hydrogen atoms to create helium, and this process generates a massive amount of energy, which produces the light and heat that make life on Earth possible.
Here on the surface of this planet, scientists are trying to replicate this process to revolutionize how we generate clean electricity. Fusion researchers believe this technology could be the key to meeting our vast energy needs.
Since fusion was discovered in 1934, we’ve been trying to harness the energy created by nuclear fusion to produce carbon-free electricity — a tremendous amount of it. The goal of fusion research is to achieve what’s called “ignition,” which is when the fusion reaction creates more energy than was used to spark the reaction.
Alex Zylstra, an experimental physicist at the Lawrence Livermore National Laboratory, said there are many other benefits to fusion — most especially that there’s no possibility of a radioactive meltdown like what might occur in a fission reactor. In a fusion reactor, “it’s very easy to stop the reaction,” Zylstra said. “If anything goes wrong, it basically just stops automatically because it’s so hard to get going in the first place.”
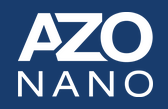

Oxygen-decorated liquid nanocarbon clusters predicted to form from shock compressed cryogenic liquid carbon monoxide. The snapshot, which is from machine-learning-driven atomistic simulations, shows only carbon (black) and oxygen (red) atoms participating in cluster formation and not the surrounding reactive fluid. Image by Rebecca Lindsey/LLNL.
They call it nanomaterial
Carbon exhibits a remarkable tendency to form nanomaterials with unusual physical and chemical properties, arising from its ability to engage in different bonding states.
Several of these “next-generation” nanomaterials, which comprise nanographite, nanodiamonds, nano-onions and amorphous nanocarbon, are being examined for potential applications encompassing fields from bio-imaging to quantum computing. Continuing research proposes that high-pressure synthesis employing carbon-rich organic precursors could pave the way for the discovery and perhaps the custom-made design of many more.
Researchers from Lawrence Livermore National Laboratory (LLNL) are keen to better comprehend how to custom-make carbon nanomaterials and how their development affects shock phenomena like detonation.
LLNL scientists conducted machine-learning-driven atomistic simulations to provide insight into the fundamental processes controlling the formation of nanocarbon materials, which could serve as a design tool, help guide experimental efforts and enable more accurate energetic materials modeling.


LLNL has provided input on Microsoft’s pathway to become carbon negative by 2030 in a new report.
A carbon removal adviser
Lawrence Livermore National Laboratory (LLNL) is working with Microsoft Corporation to map out a path for the global technology company to be carbon neutral by 2030.
Building on its 2019 report, “Getting to Neutral: Options for Negative Carbon Emission in California,” LLNL has become an adviser in Microsoft’s efforts to reduce its greenhouse gas emissions by half and removing the other half from the atmosphere through carbon storage technologies.
Microsoft has set an additional goal of removing the equivalent of the company’s past carbon dioxide emissions by 2050.
In the “Getting to Neutral,” LLNL identified a range of technologies to help California become carbon neutral, and ultimately carbon negative, by 2045. Scientists then analyzed the most promising options in that report for Microsoft to reach its goals.
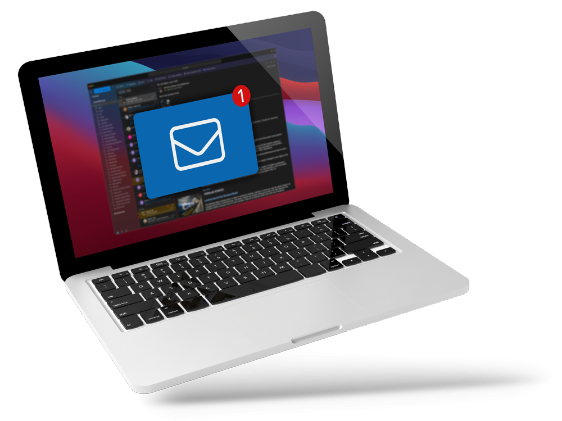