LAB REPORT
Science and Technology Making Headlines
March 11, 2022


A cryogenic target used for experiments producing burning-plasma conditions. Photo by Jason Laurea/Lawrence Livermore National Laboratory.
Go for the burn
The sun and other stars rely on gravitational compression to overcome the repulsion between atoms and power their fusion. To mimic that compression, scientists use the world’s most powerful laser at Lawrence Livermore National Laboratory’s National Ignition Facility (NIF) to squeeze isotopes of hydrogen — deuterium and tritium — in a 2-mm-wide capsule.
Conceptually, the experiment is simple: NIF trains 192 laser beams into a 1-cm-tall cylinder, known as a hohlraum, in which the capsule is suspended. In response, the hohlraum’s walls produce a flux of soft X-rays. Within eight nanoseconds, that flux compresses the capsule into a “hot spot” half the width of a human hair at a temperature of 100 million kelvin and a pressure of 350 billion atmospheres.
The NIF experiments strive to convert the kinetic energy of that implosion into internal energy faster than any other process can quench the fusion. To date, that’s been an elusive goal. In two papers, one in Nature and another in Nature Physics, the NIF collaboration reports a more modest achievement: creating a burning plasma in four experiments conducted between late 2020 and early 2021. A burning plasma is the regime in which the fusion reactions themselves — not the compression — are the primary source of heat for the plasma. And it’s an essential precursor to ignition, the state in which the heat added by the alpha particles outstrips all the losses. The resulting thermal instability triggers a nonlinear rise in temperature, which sustains and propagates the burn.
As chief scientist for the Laboratory’s Inertial Confinement Fusion Program, Omar Hurricane said: “Having reached a burning plasma, we are now on the verge of ignition.”


Dead microbial biomass in soil (microbial necromass) forms one of the largest pools of organic carbon on the planet. Different mechanisms of microbial death — such as viral lysis, predation or osmotic stress (shown above) — can influence the chemical composition of microbial necromass and its long-term persistence as soil organic carbon. Image courtesy of Victor O. Leshyk/Center for Ecosystem Science and Society, Northern Arizona University.
Wanted dead or alive
Whether dead or alive, soil microorganisms play a major role in the biogeochemical cycling of carbon in the terrestrial biosphere. But what is the specific role of death for the bacteria, fungi and microfauna that make up the soil microbiome?
That is the topic of a new review by Lawrence Livermore scientists and collaborators. The research describes how living and dead microorganisms strongly influence terrestrial biogeochemistry by forming and decomposing soil organic matter — the planet's largest terrestrial stock of organic carbon and nitrogen, and a primary source of other crucial macronutrients and micronutrients.
By shaping the turnover of soil organic matter, soil microorganisms influence atmospheric concentrations of CO2 and the global climate, as well as help provide crucial ecosystem services like soil fertility, carbon sequestration, plant productivity and soil health.
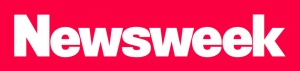

LLNL physicist James Mitrani sets up scintillator detectors to measure neutrons on the University of Washington’s Fusion Z-Pinch Experiment (FuZE) device.
Pinch me, it’s thermonuclear fusion
New research could open up another viable pathway to fusion energy, the process that powers the stars and creates their heat and light emissions.
Scientists have detected neutrons created by thermonuclear reactions in a device theorized since the 1930s. New research led by scientists from the Lawrence Livermore National Laboratory may solve a decades-old problem and show that so-called Sheared Flow Stabilized Z-pinch devices (a method of manipulating plasma a vital element of nuclear fusion) could be cured of the instabilities that disrupt fusion reactions.
The team used advanced computer modeling techniques and diagnostic measurement devices to show a sheared-flow stabilized Z-pinch device was creating neutrons as a side effect of thermonuclear reactions like those that power the stars — rather than from undesired instabilities in plasmas.
This could show these devices have the ability to generate the high-temperature conditions required to create plasma and initiate fusion within it, providing a new viable pathway to fusion power, if researchers can now get more energy out of them than it takes to kick start reactions.


LLNL researchers have proposed a new type of lens for very powerful lasers. Image credit: Adobe Stock images.
An eye on ultrahigh-intensity lasers
Two new methods for generating holographic plasma lenses capable of focusing ultrahigh-intensity lasers have been proposed by researchers in the U.S. Using computer simulations, a team led by Matthew Edwards at Lawrence Livermore worked out how the robust structures could be created by imprinting carefully generated interference patterns onto a plasma medium.
The latest advances in laser technology have allowed physicists to generate light pulses as powerful as 1015 W. However, these intense lasers are difficult to use in experiments because they cause irreparable damage to conventional solid-state optics.
A potential solution to this issue lies with plasma, an ionized state of matter that has a damage threshold several orders of magnitude higher than solid materials. Plasma has been used to generate optical components including amplifiers, gratings and mirrors — but so far, these structures have only been used to focus beams as powerful as around 1012 W.


A scanning electron microscope image of synthetic pyrite particles that was used to kill antibiotic resistant bacteria.
Fighting antibiotic resistance with nature
Antibiotics have allowed for the widespread control of bacterial infections, which had been the leading cause of death historically. However, the overuse of traditional antibiotics in humans and animals has resulted in the emergence of stronger, more potent bacterial strains that are no longer treatable with conventional antibiotics.
Researchers at Lawrence Livermore are exploring alternative treatment options when antibiotics fail. Certain naturally occurring clay deposits have been shown to harbor antimicrobial properties and kill antibiotic-resistant bacteria. These clays have been proposed as a new paradigm for fighting the potentially devastating effects of the post-antibiotic era. Despite their effectiveness, these naturally occurring clays, by their inherent heterogenous properties, exhibit variable antibacterial effectiveness and the synthesis of minerals with reproducible antibacterial activity is needed to harness their therapeutic value.
Antibiotic-resistant pathogens are predicted to account for 10 million annual deaths worldwide by the year 2050. The U.S. currently spends $20 billion a year treating more than 2 million antibiotic-resistant infections that can withstand even the most potent antibiotics. As a result, the nation's approach to medicine and agriculture will require significant changes to successfully maintain current levels of healthcare and food security.
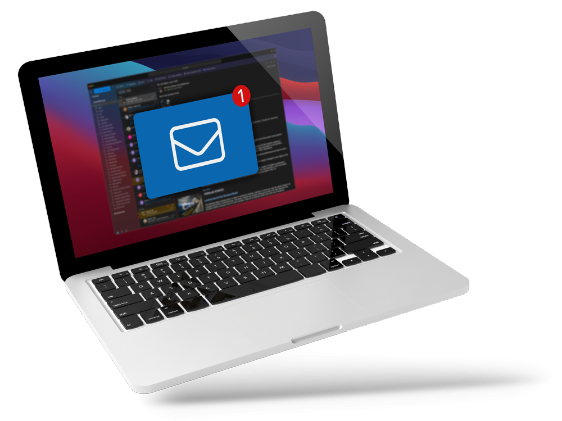